Water Reuse, Recycling, Conservation in Manufacturing
By Gregory Bachman | May 24, 2012
Category: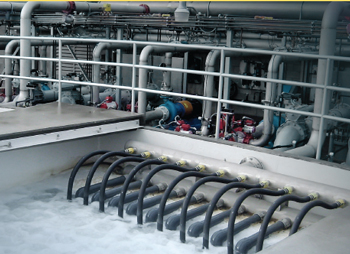
A membrane bioreactor system (MBR), which separates and treats liquids and solids, is one technology that is used in recycling water.
Water Has No Substitute. About 70 percent of the human body is water. At a 1 percent deficiency, humans sense thirst. At a 5 percent deficiency, a fever develops, and a 12 percent deficiency causes death.
There are substitutes for oil and most other natural resources, but there is no substitute for water.
Water is being withdrawn from the world’s underground stores (aquifers) many times faster than nature can replace it. The rate of net withdrawal is roughly equal to the flow of the Colorado River.
About 410,000 million gallons per day (Mgal/d) of water was withdrawn for use in the U.S. during 2005, according to the U.S. Geological Survey (USGS) Fact Sheet 2009-3098 (pubs.usgs.gov/fs/2009/3098).
World leaders and global intelligence agencies have long been forewarning that the next wars are likely to break out over access to water. As long ago as 1998, former Sen. Paul Simon referenced U.S. Intelligence reports identifying flashpoints at which war could break out over water in his book Tapped Out. More recently the U.S. Office of the Director of National Intelligence, in its report “Global Water Security,” Intelligence Community Assessment, ICA 2012-08, February 2 2012, (www.dni.gov/nic/ICA_Global%20Water%20Security.pdf) stated, “We assess that during the next 10 years, water problems will contribute to instability in states important to U.S. national security interests.”A National Public Radio program, “Will the Next War Be Fought Over Water?” explored the potential for war over water in a review of journalist Steven Solomon’s book, Water: The Epic Struggle for Wealth, Power, and Civilization.
Industry’s Impact
Agriculture is the world’s largest water consumer, according to the “Global Water Security,” report (see Figure 1), and up to 95 percent of developing countries’ water supply is used for agriculture. In the U.S., about 35 percent of freshwater is consumed by agriculture, and thermoelectric power uses 49 percent of the water supply, according to the USGS Fact Sheet 2009-3098.
Although industry consumes a lesser share, almost 5 percent of the water supply, that percentage still represents a large volume of freshwater; therefore, industry can play a major role in curbing the rate of water consumption. It may be surprising just how much industry consumes during processing. In his book, Simon cites that up to 7,060 gal. of water may be used to produce 1 ton of milk; 30,000 gal. to produce 1 ton of meat; 543,000 gal. to produce 1 ton of paper, and 27,500 gal. to produce 1 ton of recycled paper.
Even though Simon’s 1998 figures are dated, these statistics offer a glimpse of the high ratio of water use per product. In manufacturing processes, water is used to generate electric power, to make steam for processing and cleaning, to cool or control process temperature, to rinse, to dilute food, and to formulate products containing water (such as in pharmaceuticals and beverages).
How much water is required to produce a product varies, depending on how early in the process the meter starts to measure. These rates would be even higher if they included the water required to grow the crops or access the raw materials. Regardless of the method used to assess the total, clearly, industry consumes a staggering amount of water.
What Can Manufacturers Do?
You can start your own “war on water consumption” by reducing or conserving, recycling, and reusing process water. Considering that the average price of water in the U.S. is $2.81/1,000 gal., lowering consumption brings an economic benefit as well.
Progress has been made. Industrial water usage dropped from the 1950s to the late 1990s by 36 percent, while industrial production (inflation-adjusted) increased 3.7 times, according to Simon.
Recently Ford Motor Co. announced that it has set a goal to reduce its water consumption to produce a single vehicle from 2,510 gal. in 2009 to 924 gal. by 2015.
You have several tools to meet your war-on-water-consumption goals.
Conservation Methods
Conservation is defined as “keeping and protecting natural resources intact or unimpaired.” The industrial war on water consumption starts with designing and redesigning processes to use less water to begin with—doing more with less.
That can be achieved by limiting rinse steps to a meaningful specification and ending the rinse process when it carries no additional benefit to the intermediate or final product. Another way is to specify water concentration to eliminate evaporation (and the corresponding energy consumption) at a later step.
Repair Leaks. A common problem in processing plants is leaky water pipes, fittings, and valves. Often leaks occur because standard proper pipe fitting and plumbing techniques have not been used, such as using incompatible piping material and sealant compounds.
Leaks should be repaired and maintained to avoid unnecessary water loss. Even a very small leak of one drop per minute totals up to 10 gal. per year. Multiplied by all the leaks in a plant …
Clean in Place. Clean-in-Place (CIP) systems conserve water by using specially designed spray nozzles with multiple washdown steps rather than completely filling and draining.
Condensate Recovery. Other methods used for decades in boilers and power generation are condensate recovery and condensate polishing, in which condensate is reused in other process steps or for boiler feed. Not only does this reduce water consumption, it saves energy as well because the condensate is close to the required temperature.
Approach to Reuse
Reuse, defined as “to consume again” or “to make use of again,” indicates that no treatment is needed before application.
Cascading. A cascading rinse process is a good example of reuse. Final-stage rinse water that is only slightly contaminated can be used in an earlier rinse stage that does not require high purity. Then, it can be used again as grey water for coarse washing, toilet flushing, or irrigation.
Before water reuse can be implemented, however, it is critical that its effect on the process, product specification, and waste stream be considered.
Segregation of wastewater and process streams often is overlooked in manufacturing. It is far more common to combine all waste streams and deal with them downstream. An analysis of process and waste streams to determine where they can be used “as is” will reduce costs and simplify waste stream cleanup.
A complete process flow diagram of a plant–the more detailed the better–serves as a great overview of the process in determining which streams can be reused, and where.
Not all waste streams can be reused, however. Oil and grease content in a rinse or waste stream usually causes problems in final products and water systems. Even small amounts (in parts per million) can wreak havoc on reverse-osmosis and ion-exchange water treatment systems.
Recycling Technologies
Recycling water is restoring it to its original condition or to new quality specifications through a cycle of changes or treatment.
It is important to know the temperature limits on the treatment materials and equipment being deployed to recycle water. For example, the polymeric membranes used in reverse osmosis and anion-exchange resins have a maximum temperature rating as low as 110 degrees F, so process temperature cannot exceed that.
As stated earlier, it is essential to designing a water conservation, reuse, and recycling program that the final and intermediate product specifications are known and clearly stated. From this information, you can develop and implement a water specification for each step and each application.
Having access to a qualified laboratory specific to each industry, or a water lab capable of analyzing within specific levels, is critical to ensuring that a water program will be successful. Examples of the types of impurities that need to be analyzed are balanced cations and anions (i.e., inorganic salts and metals), organic impurities (generally called total organic carbon), bacteria, and
insoluble particles.
Knowing which impurities are in process water and wastewater will improve the coverage and completeness of the laboratory analysis. One advantage of reusing and recycling is knowing where the water has been and the process details. Many source water suppliers may not know or understand the impact impurities may have on a particular industry or process.
A poignant example of this is conversion of chloramines to chlorine in drinking water systems to reduce trihalomethane (THM) formation. Standard carbon filters (granular activated carbon) do not effectively remove chloramines as designed for chlorine removal. Many systems cannot tolerate chloramines and subsequent problems ensue.
Following are some common water treatment methods, in order from coarse to high purity:
Dissolved Air Flotation. This is a coarse treatment method whereby diffused air forms fine bubbles that interact with various impurities and form floating agglomerates that can be skimmed off, backwashed, or otherwise removed.
Biological Treatment. This method uses natural bacteria to break down carbon-based (organic) impurities into simpler compounds. Essentially the bacteria use the organics as a food source. The organics must have a constant level and characteristics for the bacterial species to survive in a sustainable manner.
Granular media, such as sand, or polymeric membranes, are used to attach or immobilize the bacteria so it doesn’t end up in the partially purified stream. Membrane bioreactors (MBRs) are common for such treatment.
Filtration. This is a broad category of water treatment techniques that runs the gamut from common sand filters to sophisticated ceramic and polymeric microfilters. Generally, filters physically retain undissolved particles. These particles range in size measured in millimeters to submicrons. (One micron is 1 millionth of a meter.)
Filters are designed to be backwashable layers of rock, sand, and other media; woven or pleated single-use cartridges; or crossflow ceramic or polymeric layers that concentrate the particles on the feed side of the membrane.
Granular Activated Carbon. This treatment method, also called carbon filtering, uses ashed coal, coconut shells, and other organic materials that possess unique properties to attract (adsorb) dissolved organic compounds. These typically are configured as depth filters, but also can be incorporated into cartridge filters, with limited adsorptive capacity.
The bacteria that are immobilized on the bottom portions of the carbon break down the organics as they are adsorbed on the carbon media particles. This increases the capacity of the carbon to remove organic impurities.
Carbon filters also possess catalytic properties useful for the removal of chlorine-based compounds. Typically, chlorine compounds are added to control bacterial growth, then must be removed before being used in industrial processes.
Softening. Softening is the removal of the minerals magnesium and calcium that “harden” water. This is important to eliminate scaling in boilers, heated surfaces, and parts. By far the most widely used softening technique today is cation-exchange resin operated in sodium form in a packed bed configuration. As hard water passes through media, a polymer-based resin, calcium and magnesium are “exchanged” for sodium. The media is chemically active, so it can be used for years simply by regenerating it with sodium chloride (salt).
Other methods use polymeric membranes, such as crossflow nanofilters, that possess softening properties and concentrate the hard salts on the feed side of the membrane in a resulting “reject” stream. This method eliminates salt as a regenerant chemical, but it sends more water to the drain, unless the reject stream can be used for other purposes.
Disinfection. The purpose of disinfection is to destroy and control bacteria and other microorganisms. Although chlorine compounds and other chemical oxidizers are commonplace disinfectants, where they are deployed is of key importance.
If the products being manufactured are sensitive to oxidizers, chlorine compounds must be removed. To prevent bacteria and microorganisms from surviving and proliferating, alternative disinfecting techniques can be used. Ultraviolet (UV) light systems impair and destroy bacteria at the cellular level. A combination of a UV treatment followed by very fine particle filtration and deionization effectively destroys bacteria and other microorganisms.
Deionization. Deionization is the complete removal of all ions (cations and anions). In some industries this is referred to as salt or ash removal. Two of the most common treatment methods are ion exchange and reverse osmosis.
Ion exchange is similar to softening, with an anion-exchange resin operated in the hydroxide form, with sodium hydroxide as the chemical regenerant. The cation resin must be operated in the hydrogen form and regenerated with acid (sulfuric or hydrochloric). Complete removal of cations and anions is accomplished down to levels measured in parts per billion–even in parts per trillion if needed.
Reverse osmosis (RO) is the other common method of deionization. RO consists of a high-surface-area polymeric membrane (spiral-wound is the most common) assembled in a fiberglass pressure vessel, or tube. Pretreated and pressurized water is passed into the pressure vessel. The water permeates the special selective polymeric membrane with salts being rejected on the feed side of the membrane. The purified “permeate” is collected as partially deionized water–typically 99.5 percent purified).
RO vessels are configured in arrays that both polish the permeate and recover the reject streams, passing them through a second stage to further recover more water and concentrate salts.
Getting Started
To get started on your effort to conserve, reuse, and recycle water, begin with one of your plant’s process streams. From a practical standpoint, you may want to start with a small process to “get your feet wet.”
Generally from a capital investment perspective, it makes sense to start with conservation. The key is knowing how much you can reduce your water consumption without affecting your product quality or plant performance. Next, look for reuse opportunities, and finally recycling opportunities.
A component of a water management program that is often overlooked is preventive maintenance (PM). All of the systems discussed here require varying degrees of PM and its associated costs. You should remember this in their plans, the economic justification, and budgets.
Readers who have read through to this point are no doubt interested in doing their part to save earth’s precious resource, water, and are to be congratulated for their efforts.
Case Study: Reject Recovery Reverse Osmosis Reduces Water Usage
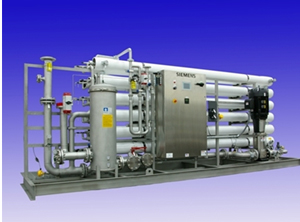
A process water treatment system implemented in 2010 at the Dr Pepper Snapple Group’s new bottling plant in Victorville, Calif., is engineered to be a sustainable and cost-effective method to meet the plant’s stringent ingredient-water specifications.
The Siemens system includes a water-saving feature known as reject recovery reverse osmosis (RO) and a backwash recovery system. The treated water is recycled for use in the manufacture of purified bottled water and various soft drinks. The system is designed to recover more than 90 percent of the water.
The 1,200-GPM system comprises media and carbon filtration, nanofiltration, RO, and UV disinfection. The Victorville plant needs nanofiltration and RO technologies because of the variety of beverages it produces and the respective ingredient-water quality requirements.
Concentrate water from the two primary nanofilters and the primary RO system is collected and sent to the reject water recovery system. The permeate from this recovery system is first-quality water and can be used for beverage production. The recovery system reduces the water sent to drain by approximately 50 percent.
Water Sustainability’s Global Reach
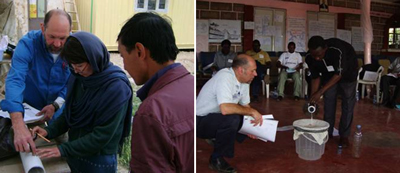
Gregory Bachman, director of technology & lab services for Evoqua Water Technologies provides water purification techniques training as a volunteer for Lifewater International.
Lifewater International is a non-profit Christian development organization that trains people in rural, remote regions of the developing world who do not have access to safe, sustainable drinking water how to access it and purify it for their daily use. Volunteers teach villagers and residents simple but effective and sustainable water purification methods—including well drilling, biosand filter construction and operation, hand pump repair, and hygiene. The training takes place in the classroom, and hands-on in the field.
According to the organization, contaminated water is a major source of disease and death in the developing countries. Every day 4,000 children die of water-related diseases. Nearly one in five child deaths—about 1.5 million each year—is due to diarrhea. In developing nations, about 90 percent of sewage systems are being emptied into rivers, lakes, and nearby streams that communities use for drinking water. The combination of access to safe water, sanitation, and hygiene education reduces the incidence of diarrheal disease by 60 percent.
Providing people with a way to tap clean water, sustainably, literally is a life-and-death matter.
Lifewater International, www.lifewater.org
Side by side, we move metal fabrication forward.
FMA unites thousands of metal fabrication and manufacturing professionals around a common purpose: to shape the future of our industry, and in turn shape the world.
Learn More About FMA